Membraneless Soluble Lead Redox Flow Cell at Low to High External Flow Rates
Renewable energy sources such as solar and wind are intermittent in nature. Their effective utilization requires efficient, low cost energy storage systems to complement energy harnessing systems. The rechargeable flow batteries have emerged as the most suitable candidates due to their low cost per kWh, long cycle life, and low maintenance. The electrical energy is stored as chemical energy through reversible redox reaction when electrolyte flows through or over the electrodes. While the vanadium redox flow battery, which requires periodic replacement of expensive proton exchange membrane, is currently the most promising one, the soluble lead redox flow battery (SLFB) is potentially among the least expensive ones in its class, because of the raw material used and the membraneless single electrolyte flow loop. The understanding of the battery is in its early stages. The major challenge faced in taking soluble lead redox chemistry to energy storage technology is to increase its cycle life.
In the present work, we report our investigations on the effect of electrolyte flow rate on charging characteristics of an inhouse constructed SLRFB cell, shown schematically in Fig. 1a. The electrolyte flow rate is a critical parameter in vanadium flow battery as low flow rates lead to high charging over-potential and high flow rates through porous electrodes lead to high pressure drops. Fig. 1b shows the experimentally measured effect of flow rate on the variation of charging potential with time for our cell. The figure shows that a 30 fold decrease in flow rate to extremely low values also does not increase the overpotential required to charge the cell. Furthermore, the measurements do not show transient effects either, except for an initial peak that is attributed to the birth of nuclei of PbO2 and Pb on anode and cathode respectively. We carried out full-scale COMSOL Multiphysics isothermal simulations, that required a solution of unsteady-state two-dimensional fluid flow equations, potential field, species transport, and electrodeposition/electro-dissolution reactions involving Pb++ ions in the electrolyte.
Figure 1c shows the model predicted cell potential during charging. The figure shows that the model captures the experimental observations very well. The charging potential remains nearly constant with time, and independent of flow rate down to 1 mL/min. The simulations show (figure not added here) that in the absence of natural convection, even at an external flow rate of 5 mL/min, the cell does not charge beyond 100 seconds. The overpotential required becomes unacceptably large. The reasons for the dominant role of natural convection, while the external flow rate is varied over 30 times, will be highlighted in the presentation along with the demonstration of the potential of split-electrode designs to harness natural convection.
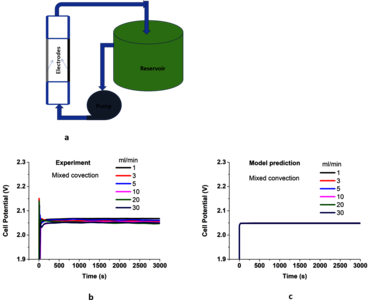