Modeling Transport of Antimicrobials Encapsulated in Yeast Microparticles for Biofilm Inactivation
A biofilm is a community of microbes embedded in a self-secreted polymer matrix. It may persist in different environments, and in the case of pathogens, these biofilms can be a significant risk factor for foodborne disease and healthcare-associated infections. Due to the unique structure and compositional features, it is often challenging to inactivate bacteria in biofilms. These challenges include the consumption of antimicrobials by the biofilm matrix as well as enhanced resistance of bacteria in biofilms. To address these challenges, we are developing bio-based carriers that have a specific affinity to bind biofilms, resist non-specific consumption of antimicrobials, and maintain persistent inactivation activity against bacteria in a biofilm. This study focuses on modeling chemical reaction kinetics and transport phenomena to characterize enhancement in inactivation efficacy of antimicrobials using bio-based carriers. For developing the model, chlorine was selected as one of the common antimicrobials and yeast cell microparticles (YMPs) were selected as bio-carriers with affinity to bind biofilms. Escherichia coli O157:H7 biofilm was used as a model pathogenic biofilm. In our model systems, local binding of YMPs to E. coli biofilm and chlorine release from YMPs were characterized mathematically and then combined with the mass transport in wash water as well as biofilm (porous media). The binding affinity and release kinetics were experimentally measured and fitted to the pseudo-first-order and first-order reactions, respectively. This system of equations was implemented in COMSOL Multiphysics® software and solved for various conditions. The model geometry was defined as an axisymmetric cylinder, mimicking the actual system in experimental validation. Three different Transport of Diluted Species interfaces were used to solve for the distributions of YMPs in the wash water, chlorine in the wash water, and chlorine in the biofilm, respectively. Moreover, Boundary ODEs and DAEs was applied for the mass conservation during the surface binding of the YMPs. The adaptive mesh was used to model the boundary layers of the biofilm. The simulation results indicate that free chlorine was instantaneously depleted when exposed to the wash water with organic content, while encapsulation of chlorine into YMPs significantly enhanced its stability. Consequently, E. coli in the biofilm survived against free chlorine added to the wash water, but they were efficiently disinfected when chlorine in YMPs was used in the wash water. The simulated biofilm inactivation efficacy results were in agreement with our previous experimental observation. In addition, different rate parameters were explored for the binding of YMPs and the release rate of encapsulated antimicrobials. The parametric evaluation illustrated that binding affinity is the dominant factor in improving the targeted delivery of chlorine to the biofilm and for achieving rapid inactivation of bacteria in a biofilm. The numerical simulation results were further validated by experimental measurement using bio-based carriers with differences in binding affinity to a biofilm matrix. Overall, the results in this study illustrate the potential of a multiphysics modeling approach to enable the rational design of anti-microbial delivery approaches for the treatment of biofilms.
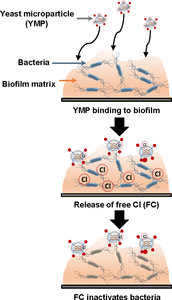
Download
- J_Yi-2020Comsol_poster_final.pdf - 0.47MB