Numerical Model of Induced Transmembrane Voltage for Cell Electroporation Using COMSOL Multiphysics®
Electroporation is the process of creating temporary hydrophilic pores in the cell membrane by applying an external electric field of specific strength and duration. It is used for gene transfection, and transport of other useful biomolecules and therapeutic drugs into the cell. When a sufficiently large enough electric potential difference is induced across the poles of a spherical cell due to the electric field, cell membrane electroporation occurs. Microfluidic devices are used to carry out single-cell electroporation with high accuracy and greater cell viability. The proper understanding of the electric field distribution and cell membrane permeabilization is a key factor for the creation of such platforms. Finite-element analysis of a spherically shaped biological cell to compare the differences in induced transmembrane voltage (ITV) of a cell electroporation model using two different approaches is presented in this work. The cell membrane was defined with a boundary condition in one model and a full-fidelity model with a physical thickness in another. The electric field distribution generated from the two models were compared to each other and with the analytical solution for ITV.
The modeling software— COMSOL Multiphysics®, with an external field of 1kV/cm and a cell membrane thickness of 5nm, was used to develop the computational cell-electroporation model. The Electric Currents interface in the AC/DC Module with dense-customized meshing and a time-dependent study approach was used for defining the models. The variation in induced transmembrane voltage for each modeling approach was investigated using post-study result analysis and MATLAB® tools. A good agreement with the analytical results for ITV was found for both the models. The limiting condition of zero conductivity was analyzed and it was proven that the equation collapses to ITV=1.5ERcosθ irrespective of the cell-membrane defining approach. The two opposite poles of the cell membrane closest to the electrodes underwent poration due to the electric field concentration in those regions and resulted in ITV values of approximately ≥ 0.5V. On the anodic and cathodic poles of the cell membrane for both the models, a considerable drop in the induced transmembrane voltage was observed because of the increased permeability due to pore formation. On adding the steady resting transmembrane voltage of the cell (-70mV) to the ITV calculation, the anodic side became hyperpolarized and the cathodic side became depolarized for both the boundary condition and the full-fidelity models.
These results indicate that the two different approaches to defining the cell membrane produces comparable values of total transmembrane voltages before and after electroporation, with only differences in their meshing and material-defining techniques. These studies can be translated into appropriate model selection for studying single-cell electroporation using microfluidic devices.
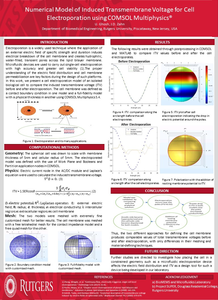