Numerical Modeling of 3D Electrowetting Droplet Actuation and Cooling of a Hotspot
Electrowetting on Dielectric (EWOD) is a versatile tool for performing basic operations in Digital Microfluidic Systems. In EWOD, surface tension between a liquid droplet and solid substrate is modulated by applying voltage to an electrode and a hydrophobic surface is transformed into a hydrophilic one. Thus a droplet partially placed on the activated electrode can be moved due to asymmetric electrowetting force. Among many applications of EWOD reported in the literature [Ref. 1], a novel technique is hotspot cooling for more efficient and target specific thermal management of electronics. In this technique, a programmable array of discrete microdroplets can be used to actively cool areas of locally high heat flux known as ‘‘hot spots.’’ [Ref. 2 & 3]
In our present study, COMSOL Multiphysics® software has been used to model the coupled droplet transport and hotspot cooling phenomena. The Navier-Stokes equation has been solved for modeling three dimensional water droplet actuation in air medium using both the Two-phase Flow, Phase Field and Two-phase Flow, Level Set interfaces and each has been coupled with the Heat Transfer in Fluids interface to model hotspot cooling. Figure 1 shows the model setup. Instead of coupling electrostatics with two-phase flow physics, electrowetting effect has been incorporated into the model by using wetted wall condition in two-phase flow interface on the electrode surface which includes modified contact angle defined by Young-Lippmann equation expressing the relation between the applied voltage and change in contact angle. To imitate the heat generation in a rectangular hotspot, a constant heat flux condition has been considered in Heat Transfer in Fluids interface.
Our preliminary results are promising and show good agreement with experiment. Figure 2 shows consecutive positions of the droplet during transport. Figure 3 compares the results of the droplet profiles at different time instants from simulation with that of the experimental droplet profiles at the same time instants. This is a validation of our simulation since droplet profiles from simulation and experiment show close match. In the non-conservative phase field method, the droplet mass is not completely conserved which is shown in Figure 3 and in the conservative Level Set method, droplet profile is slightly different than the experimental case and computationally very time consuming. So currently both of these problems are being investigated for improvement. Figure 4 shows the average hotspot temperature. The cooling curve from multiphysics simulation shows that heat is removed from the hotspot by the droplet and the trend of this cooling curve matches with that observed in the experiment.
More detailed simulations are being carried out at present for different experimental parameters. Measuring temperature and other heat transfer parameters such as heat transfer coefficients at numerous locations experimentally are required to obtain the cooling capacity of hotspot droplet cooling and also to find out the parameters for optimization towards better cooling performance. These experimental measurement techniques make the system technically more complicated and cumbersome. This simple coupled fluid flow and heat transfer model can be very helpful in this situation.
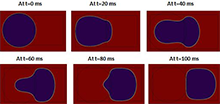
Download
- nahar_presentation.pdf - 1.24MB
- nahar_paper.pdf - 0.6MB
- nahar_abstract.pdf - 0.03MB