Optimizing your Femtosecond Laser Processes using a Numerical Simulation based Decision Support Tool
Laser surface texturing has emerged as a promising texturing technique due to properties such as excellent repeatability, non-contact process, the ability to achieve small-size features and high-quality finishing. The work described here is part of the H2020 research program called SHARK which aims at developing laser surface texturing from the current trial-and-error, lab-scale concept to a highly predictable, finite element (FE) modelling and data driven industrial approach. One of the challenges of the SHARK project is to overcome the lack of knowledge and resources available to inform the laser parameters selection.
Femto-second laser texturing offers the possibility to reduce significantly the amount of molten material. This means better surface properties as well as more accurate prediction of the surface topography. In this paper, a model that captures the important physical phenomena involved in the femto-second laser texturing process is developed and presented. The ultra-short deposition of the energy is taken into account in this model through an approach called the “two-temperature model”, which consists in modelling the heating of the component through two phases. During the pulse duration, only the heating of the electrons is computed, as the timescale for continuous energy transfer is not reached. Then the heat transfer from the electron to the lattice of the material is modeled by solving the energy equation in the component. A coupling term between the temperature of the electrons and the temperature of the lattice is included to account for the heating of the lattice from the electrons energy release. The interest to use this model was demonstrated for pulse durations from several tens of femtoseconds to several tens of picoseconds [1]. The flux of ablated matter is computed using a convective heat flux under the hypothesis that the surface does not exceed significantly the vaporization temperature. The mesh is then moved by computing the mass flux leaving the liquid-gas interface from the heat energy lost by vaporization. The results obtained from this model are compared with existing data from literature [2]. The model can then be used to study the influence of laser parameters (average power, pulse duration, frequency…) on the final topography.
This project has received funding from the European Union’s Horizon 2020 Framework Program for research and innovation under grant agreement no 768701.
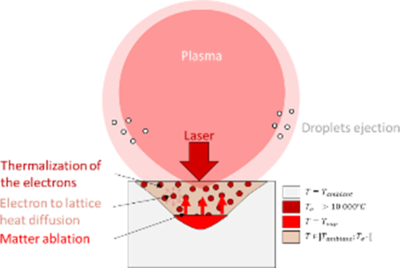
Download
- Chevallier_posterSHARK_ConfCOMSOL_2020.pdf - 0.38MB
- Chevallier_Laser_ConfCOMSOL_20.pdf - 0.35MB