Simulation of a Piezoelectric Catheter-Based Acoustic Ablation Device
Thermal ablation driven by an acoustic heat source has tremendous potential for the treatment of diseased tissue. In many applications, such as treating tumors in the liver, bulk removal of tissue is required, and thermal ablation can reliably cause necrosis for a relatively large tissue volume by inducing coagulative necrosis. Catheter-based acoustic ablation devices have great potential to be used for the treatment of cancer in regions that are surgically inoperable. Using COMSOL Multiphysics®, we developed a model that simulates the generation and propagation of acoustic waves through tissue using a piezoelectric catheter-based tissue ablation device. The purpose of the model is to help understand the interaction between acoustics and heat transfer necessary for a minimally invasive acoustic ablation device. We modeled a catheter ablation system with an array of piezoelectric acoustic transducers based on an existing catheter design, shown in Figure 1(a). The model included the ablation device, a coolant surrounding the device, the catheter, and the tissue to be ablated. The model accounted for the piezoelectric coupling between electrostatics and solid mechanics in the piezoelectric transducers. We used solid mechanics coupled with pressure acoustics to model the harmonic pressure variation in the system. We modeled the coolant flow within the catheter and around the ablation device using laminar flow. We accounted for the losses from the pressure field as an acoustic heat source in a transient heat transfer model including the bioheat equation. We investigated how changing parameters such as the number of piezoelectric transducers, the temperature of the coolant, and the distribution of power within the piezoelectric transducers affected the ablation zone and heating temperature. We also determined the fraction of tissue damage using an Arrhenius model based on the time the tissue was exposed above a certain temperature threshold. From the temperature distribution and tissue damage results, we were able to investigate how design and input parameters could be changed to optimize ablation device performance and produce a targeted heat source that would be most effective in minimizing tissue damage outside the ablation zone. Figure 1(b) shows the acoustic pressure variation in the device and surrounding tissue for one set of operating conditions.
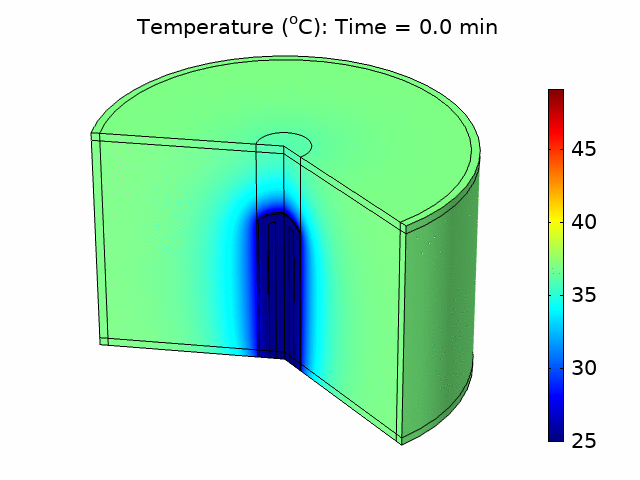
Download
- M-Gobran-Acoustic Ablation Presentation.pdf - 0.79MB
- Acoustic Ablation Paper.pdf - 0.41MB