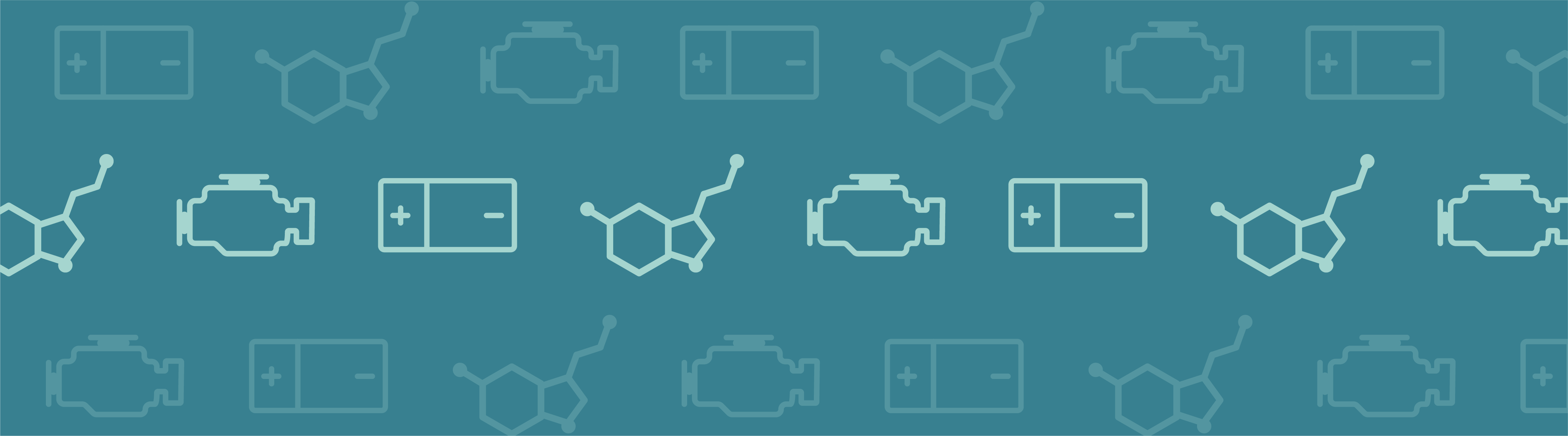
Guest blogger Bojan Jokanović of SGL Carbon GmbH, one of the world’s leading manufacturers of carbon-based products, discusses the optimization of thermal processes in the carbon industry.
Carbon products are used in many industries, including semiconductors, car manufacturing, ceramics, and metallurgy. Properties of graphite including high-temperature stability, good thermal and electric conducting behavior, and high chemical stability make this material unique. However, carbon manufacturing is an energy-intensive industry. We must build digital process chains to optimize processes and minimize costs.
The Manufacturing Process of Carbon Products
Typically, carbon products such as graphite are made from raw materials such as petroleum or coal tar pitch coke, pitch as a binder, and some additives. To enable a dense material structure and to avoid large defects in the material, it is crucial to optimize the recipe of all fractions, too. After mixing, the next step is forming; e.g., extrusion, isostatic pressing, or similar shaping processes.
The graphite manufacturing process.
The material is consequently baked at temperatures of 600–1000°C, where the pitch is pyrolyzed. Some gases, like methane, hydrogen, and steam, evolve during this process. As the process begins, the pitch melts and the material becomes viscoplastic and vulnerable. At this process step, the material has very low stiffness and strength, and the gas pressure coming from the pyrolysis can cause deformation and cracks. After baking, the material is hard and contains a porous structure that is, in the next step, typically impregnated either by resin or pitch. The time for the impregnation depends on the product permeability and the autoclave conditions, pressure, and temperature. Such impregnated products are again pyrolyzed and finally graphitized.
Graphitization is a high-temperature process where temperatures of 3000°C are achieved. During the graphitization process, the material is typically exposed to a high thermal load, mostly through electric heating and commonly through Joule heating. In this step, the graphite crystal structure is obtained and the material becomes electrically and thermally conductive as well as soft and easily machinable.
Simulating Thermal Processes for Carbon with COMSOL Multiphysics®
For the simulation of most of these processes, we use the COMSOL Multiphysics® software, since it gives us the flexibility to implement our own models and its scaling and parallelization functionality enable fast calculation of these phenomena.
Consider the baking part of the graphite manufacturing process. We use chemical reaction kinetics to calculate the gas evolution rate. The gas needs to be transported through the material subject to Darcy’s law.
where \mathbf{u} is the fluid superficial velocity; p is the pressure; \varepsilon is the porosity; {Q_\textrm{m}} is the mass source term (in our case, created gas quantity); \kappa is the material permeability; and \eta and \rho are fluid viscosity and density, respectively.
The gases are created in Arrhenius-type kinetics:
where {k_{\textrm{0}i}} is the pre-exponential factor, {E_{\textrm{A}i}} is the activation energy, {n_i} is the reaction order, R is the universal gas constant, T is temperature, and {c_i} is the reactant concentration.
As a consequence, the gas evolution in the material will be affected through the heating rate. The higher the heating rate, the faster the gas evolution. However, if more gas is created than conducted through the material pores, as described by the material permeability, then the pressure will grow and cause stress and potential damage in the material.
The properties are temperature dependent and we evaluate them using experiments in our in-house laboratory. The gases are considered to behave according to the ideal gas law. The material goes through structural changes that are characterized by changes in material properties, such as thermal conductivity, the coefficient of thermal expansion, stiffness, and more. The thermal stress will also exist due to inhomogeneous temperature distribution, causing inhomogeneous structure change and thermal expansion behavior. This thermal stress contributes to the stress coming from the gas and we optimize our baking programs to stay below the limits required for optimal product quality.
In the simulation of the graphitization process, we must consider the material changes and the restrictions given by the equipment. Typically, the material’s resistivity decreases tenfold during the process and the rectifier is pushed to its limits. At the beginning of the process, we usually have the maximum voltage limitation. At the end of the process, since the material becomes much more electrically conductive, we achieve the rectifier limit for maximum current. Besides, we must take care of the mechanical stresses, especially since this is the final thermal treatment and the costs of scrap are very high. COMSOL Multiphysics gives us an opportunity to implement these restrictions.
In our graphitization model, we use Joule heating physics together with the thermal stress calculation. Besides the anisotropic and temperature-dependent material properties like thermal conductivity, electrical resistivity, and coefficients of the thermal expansion, we can also measure high-temperature stress-strain behavior in our lab, and we implement all of these properties in our model. For the evaluation of stresses, we developed our own stress criterion based on the combination of the principal stresses and the strength of the material along the process.
Left: The graphitization furnace (double-mirrored view). Right: The automatic furnace control based on the implemented restrictions. In each time step, all of the restrictions are recalculated and based on the logical expression result, and one of them is selected.
Helping Customers with Simulation
Not only do we model our processes using COMSOL Multiphysics, but we also use simulation to support our equipment purchases and to analyze customer processes. This helps to better understand customer product requirements and offer them the best value for their money. We also offer our customers modeling and experimental validation in our in-house lab.
The image below shows the plurality of the SGL Carbon products employed in high-temperature applications. Typically, simulation can help us determine the right combination of the insulation materials, calculate the temperature within the furnace, and minimize the heat losses through joints. Finally, the mechanical stability and thermal load must also be tested and the heaters and geometry of the charging racks must be optimized.
SGL Carbon GmbH products for high-temperature applications, CFRC, and graphite heaters: a CFRC charging rack, hard graphite-felt insulation with radiation-minimizing graphite foil, and a CRFC fan. Courtesy SGL Forum Exhibit, Meitingen, Germany.
Due to the multiphysics capabilities, we can extend the model in COMSOL Multiphysics to include induction heating, like in the 3D induction heating example given below. We can calculate the eddy currents in the susceptor, objects within the furnace, temperature homogeneity, and energy losses within the insulation and coil. We can also optimize the circuit frequency to enable the most efficient coupling. At the same time, we calculate the heat transfer through conduction and radiation and make sure that the load is heated homogeneously.
A bell jar induction heating furnace with a spherical load.
Using Simulation Apps for Technical Sales Support
When it comes to customer consulting, simulation is gaining importance and COMSOL apps offer a simplified interface with the possibility to calculate some frequent problems. For instance, graphite heaters can be calculated with a different number of segments, filleted or nonfilleted edges, manipulated furnace power, and hard or soft felts. This gives us a first impression of the temperature and stresses in the graphite heater and felt. Certainly, professional skills are needed to make a robust model that converges for a wide range of boundary conditions and runs in the background of the app. The users, who are not familiar with the modeling aspect, don’t have to bother with it and can use the app without difficulties.
Left: An app for the graphite heater insulated with graphite felt. Right: A felt calculation app.
In the second presented case, the technical sales team advises customers about proper insulation assembly. The app enables the selection of felts and support, and the user can vary the number of layers and their thickness according to customer limitations. Users can also set the furnace temperature. The app calculates the heat losses through the insulation and the outer temperature of the felt. The proper boundary conditions on the outer side are automatically set. The modeling group in charge of central innovation offers unique expertise within modeling as a consultancy service to external customers in many industries.
About the Author
Bojan Jokanović works in the modeling group of SGL Carbon GmbH, Germany. He obtained a PhD from the Technical University in Clausthal, working on the modeling of the oxidation of coated carbon composites. His first experience with COMSOL was in 2002, when he started with the simulation of heat transfer during cyclic loading. His main professional interest and specialization is in the fields of process modeling and simulation, as well as optimization, both of physical and business processes.
Comments (0)