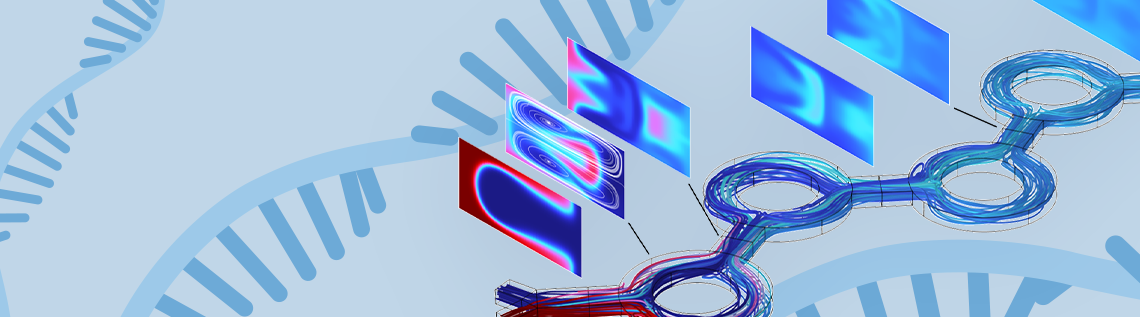
Lipid nanoparticles (LNPs) are used in a variety of pharmaceutical applications, including viral vaccines, cancer therapies, analgesics, and photodynamic therapies. In recent times, they have gained public attention for their use in mRNA vaccines, specifically. The mRNA vaccine delivery efficacy depends on the size of the LNPs and dosing: small LNPs are better at penetrating tissue and high doses are required due to low delivery yield. Researchers could run iterative experiments to meet these requirements, but a team from Veryst Engineering proposed that a simulation-guided design process would complement the experimental work and could ultimately save on cost and time as well as aid in finding more innovative solutions.
mRNA Vaccine Production: Mixing and Self-Assembly
Veryst Engineering is an engineering consulting firm that specializes in the use of simulation and analysis for product design, manufacturing processes, and failure analysis. In a keynote talk from COMSOL Day: Pharmaceutical Applications, Matthew Hancock, a partner at Veryst, and Joseph Barakat, a senior engineer at Veryst, gave an introduction to the production of mRNA vaccines and shared how simulation can enhance the design process behind these nanomedicines.
“Broadly speaking, what you have [in mRNA vaccine production] is an organic phase being mixed with an aqueous phase. The aqueous phase contains the mRNA, which is negatively charged, and the organic phase contains the lipids that are meant to encapsulate it,” said Barakat. “Given sufficient residence and mixing time, these components mix and self-assemble to spontaneously form aggregates.” It is these aggregates, the LNPs, that make up the mRNA vaccines that have captured public attention in recent years.
Figure 1. A schematic detailing the production of an mRNA vaccine. Image courtesy of Veryst Engineering.
Barakat explained that there are two common ways that the components can be mixed to form these aggregates. For production-scale drug manufacturing, turbulent flows are used, which rapidly mix via the cascade of large eddies breaking down into smaller and smaller eddies, which enhances the effectiveness of molecular diffusion. For small-batch drug manufacturing, such as that employed in drug discovery and development or for personalized medicine, microfluidic devices are employed due to their low fluid volumes. “The challenge is that with microfluidic devices […] in order to achieve efficient, rapid mixing, you cannot take advantage of the turbulent mixing, which is very efficient,” said Barakat. Moreover, even though such devices are small, molecular diffusion is generally speaking still far too slow to achieve the desired rate of mixing. However, there are several active and passive forms of mixing that are appropriate in microfluidic devices, including chaotic mixing.
Understanding which mixing method is best for making mRNA vaccines is an important first step. When it comes to creating the vaccine, there are additional challenges to overcome.
The Challenges of Lipid Nanoparticle Production
Producing mRNA vaccines is a difficult task. The size of the LNPs (which directly affects the efficacy of these nanomedicines) is largely dependent on mixing time. “In general, longer mixing times give lipids more time to aggregate, leading to larger aggregates and more heterogeneous size distributions, while shorter mixing times lead to smaller nanoparticles, but with lower yield,” said Hancock.
Figure 2. Two bottled mRNA vaccines. Photo by Spencer Davis on Unsplash.
The fine-tuning of mixing time may be achieved through iterative experimentation, but producing and testing real-life devices is time consuming and costly. Simulation can complement the experimental design process for both small-batch and production scale processes. In the keynote, Veryst presented an example where simulation was used to compare different microfluidic device designs that achieved chaotic mixing via geometric features.
Chaotic Mixing in Microfluidic Devices
In the keynote, Hancock outlined mixing predictions for three microfluidic device designs which have been reported to produce LNPs in real-world experiments. In each design, ethanol, which carries the lipids, flows from one inlet, while water, which carries the mRNA molecules, flows from another. The two fluids then merge and progress together through each device. Ideally, all three device designs should allow the ethanol and water to mix well and the components to self-assemble into LNPs along the way. The success of this process is shown by the predicted streamlines and ethanol concentration in successive cross-sections along the channels (Figures 3–5).
Model 1: Serpentine Mixer
In the first model (Figure 3), Hancock analyzed a microfluidic device with a serpentine design. “The microfluidic serpentine mixer achieves efficient chaotic mixing through the eddies generated by the serpentine channels,” said Hancock. “The channel height on this device is 100 microns which is typical of microfluidic devices.”
In this design, at high flow rates (Reynolds numbers of 20–100), the ethanol and water are completely mixed by the end of the serpentine channel, which is desirable for LNP formation and for producing mRNA vaccines. In Figure 3, “the sequence of images show the ethanol concentration distribution in channel cross sections and shows how the mixing progressively occurs along the channel,” said Hancock. Unfortunately, at lower flow rates, this device was incapable of producing the eddies and inertial effects needed for good mixing and efficient LNP production.
Figure 3. Streamlines and ethanol concentration in channel cross-sections along a microfluidic device with a serpentine mixer design. Image courtesy of Veryst Engineering.
Model 2: Staggered Herringbone Mixer
The second model (Figure 4) was of a staggered herringbone mixer. “It is one of those mixers that when people see it, they remember it,” Hancock said. The design consists of “herringbone-shaped grooves that alternate in orientation along the bottom of the channel to cause a type of rifling of the streamlines.”
The alternating orientation of these grooves promotes a Baker’s transformation on the contents of the channel. Or, as Hancock explained, “It takes these initially large chunks of two different solutions and splits and recombines them to progressively produce more and more alternating layers of each solution that are closer and closer together.” As the layers of the two solutions get closer together, they mix more rapidly by the process of molecular diffusion. The herringbone mixer provides effective mixing across flow rates, that is, without the high flow rate limitation of the serpentine design.
“It turns out that this particular type of mixer is efficient across a wide range of flow rates and Reynolds numbers,” said Hancock. This model’s predictions imply that, when fabricated, the herringbone mixer should be effective at producing LNPs and mRNA vaccines.
Figure 4. Streamlines and ethanol concentration in channel cross-sections along a microfluidic device with a staggered herringbone mixer design. Image courtesy of Veryst Engineering.
Model 3: Ring Mixer
The final model discussed in the keynote was of a microfluidic mixer comprising a sequence of ring-shaped channels. “[This] type of microfluidic, chaotic mixer uses Dean flow,” said Hancock. “Dean flow is a type of circulation that develops as fluid moves around a curved channel and it is active at higher flow rates and Reynolds numbers.”
The fluids were mixed relatively successfully in the simulation of this model, but, like the serpentine approach, mixing required higher Reynolds numbers. Hancock also noted that even though the degree of mixing improved after moving through each ring, the pictured design (Figure 5) would require more rings to produce ideal mixing.
Figure 5. Streamlines and ethanol concentration in channel cross-sections along a microfluidic device with a ring mixer design.
Combining Simulation with Lab Experiments for Better Designs, Faster
With simulation, Barakat and Hancock were able to test the effectiveness of different microfluidic, chaotic mixer designs and optimize the typically slow process of microfluidic mixing. Ideally, such simulations and design optimizations would be initiated before fabricating experimental mixer prototypes. Using simulation before building prototypes increases the likelihood that prototypes will perform well and reduces the number of prototypes that need to be built — all resulting in time and cost savings. Within this work, simulations indicated that the design of the ring mixer should include more rings and how mixing performance depends on operating parameters, namely flow rate and Reynolds number. For the production of LNPs and mRNA vaccines, lab experiments would be needed to test the size distributions of the LNPs formed in each mixer design, which could then be correlated to mixing metrics.
As shown throughout their talk, multiphysics simulation of flow, transport, and LNP self-assembly can be used to:
- Improve the understanding of flow and kinetic limitations to LNP formation
- Relate key outcomes with system parameters
- Provide quantitative estimates of meaningful intermediate and final quantities (such as mixing times and population size distribution)
- Reduce the number of costly lab experiments and increase the value of each experiment that is run
- Guide the design iteration path and offer new avenues for development
“We have shown that effective mixing can be achieved in microfluidic devices,” said Hancock. “Simulations can help tune the design parameters to optimize performance prior to fabrication and testing.”
Next Step
Watch the recorded keynote presentation from Veryst Engineering to learn more about chaotic mixing in microfluidic devices and see how they model the coupled mixing and LNP self-assembly involved in mRNA vaccine production.
About Veryst Engineering
Veryst Engineering, LLC provides premium engineering consulting services at the interface of technology and manufacturing. They aim to be the best in the world for multiphysics simulation, materials science, failure analysis, and material modeling and testing, often applied to nonlinear, coupled problems, so that their clients can deliver the best products to their customers. They help clients around the world optimize product design, improve manufacturing processes, and diagnose product problems.
Comments (0)