Producing Power, Protecting Fish with a Darrieus Water Turbine
The Netherlands employs coastal flood control structures that could also be used to harness tidal power. Strict national and European regulations drive new turbine designs that are inherently safe for fish passage. To develop such a rotor design for client Water2Energy, Physixfactor used simulation to adapt a Darrieus wind turbine for water use. Compared to conventional hydropower turbines, experiments show that the Water2Energy vertical-axis design reduces fish mortality rates from 20% to less than 1%.
By Alan Petrillo
April 2021
“Here in the Netherlands, we are pretty close to the sea,” says Helger Van Halewijn, who then smiles at his understatement. The North Sea (Noordzee) and the Dutch are inseparable. From this intimate and turbulent relationship, the people of the Netherlands have learned to be resilient, and also flexible. Rather than fighting with the water, it has long been wiser to negotiate a wary truce. The famous Dutch landscape of dykes, canals, and polders does not stop the sea, so much as it redirects water flow into something manageable — and useful.
This resourcefulness lives on in modern Dutch infrastructure projects and in the people who make them possible. “We not only want to use our dykes for flood protection. We can also use them to address the need for energy and to protect fish and the environment,” says Van Halewijn, the director of engineering design consultancy Physixfactor. To achieve these goals, Dutch company Water2Energy turned to Van Halewijn to support the modeling of their vertical-axis water turbine (VAWT) for use in flood-control structures. Using multiphysics simulation, he optimized the Water2Energy VAWT to produce more electric power while minimizing potential harm to sea life.
The technology of this tidal power project may be modern, as is its emphasis on environmental protection, but its roots run deep into the vulnerable (but carefully guarded) Dutch soil.
Zeeland and the Delta Works: Protecting a Precarious Place
If any region of the Netherlands can be considered closest to (and most affected by) the sea, it may be the section with “sea” in its name: Zeeland. The westernmost and least populated Dutch province is also a river delta, where the Scheldt, Meuse, and Rhine all flow into the North Sea. More than one third of Zeeland’s total area is water. Its Latin motto of luctor et emergo, or “I struggle and emerge,” is illustrated on Zeeland’s coat of arms by a lion rising out of the waves.
Even by Dutch standards, Zeeland is especially vulnerable to North Sea storms, and the 1953 storm known today as Watersnoodramp permanently reshaped the region. A combination of winds, tides, and storm surge caused the sea level to rise more than 4 meters above average, breaching dykes and inundating 165,000 hectares of land. More than 1800 people were killed and tens of thousands were forced to flee the area. The Netherlands responded by building a sophisticated system of dams and barriers throughout the delta region.
In the Dutch tradition, these Deltawerken, or Delta Works, did not completely wall off the sea from the land. The need for protection against periodic storms had to be balanced against the region’s everyday needs, including fishery and river access to the major ports of Rotterdam and Antwerp, Belgium. Therefore, the Delta Works combined some fixed barriers with other semiopen structures, closing only when there is a threatening storm surge.
Feedback Loop: The Evolving Priorities of the Delta Works
As would be expected from such a large and complex project, construction of the Delta Works has lasted for decades (Figure 1). The project’s priorities have continued to evolve over the 70+ years since it began. Along with providing protection from sea storms, the Delta Works have also changed the regional ecosystem — not always for the better. “In the 1950s and ‘60s, when the project was designed, it was completely new. Nobody in the world had done this type of waterworks before,” explains Van Halewijn. “Concern for the environment was not like it is today.”
Aside from the semiopen barriers mentioned above, the original Delta Works included dams that blocked off some estuaries. This created new boundaries between salty seawater and freshwater from the rivers. Behind the dams, areas that had previously been subject to tidal action instead became freshwater lakes. “Nowadays we see that was a mistake,” Van Halewijn says. Since the 1970s, sluices have been installed in a number of dams. These controlled passages are kept open under normal conditions and are closed only during storms. By reintroducing tidal cycles to the basins behind the dams, the sluices have restored the salty conditions preferred by oysters, mussels, and other coastal sea life.
ENCORE and Water2Energy: Renewable Energy from Vulnerable Places
While Zeeland’s circumstances are unusual, rising sea levels caused by climate change are threatening coastal regions worldwide. The infrastructural expertise of the Netherlands, learned from many centuries of bargaining with the sea, is more globally relevant than ever before. In this context, it is no surprise to see the Dutch lead cross-border initiatives like Energizing Coastal Regions with Offshore Renewable Energy (ENCORE).
A joint project funded through the Interreg 2 Seas program and led by marine renewable energy expert BLUESPRING, ENCORE recognizes the North Sea region’s vulnerability to climate change, as well as its potential as a source of energy production. The project, with partners from the U.K., France, Belgium, and the Netherlands, affirms that 25% of European energy demand could be met with offshore renewable sources by 2050. Three participating companies are developing offshore solar power, wave energy technology, and a river turbine. The third company, Water2Energy, seeks to produce power from the tidal flows through Delta Works sluices.
The Darrieus Rotor: Adapting a Wind Turbine for Water
A place defined by the restless movement of water seems like an ideal setting for hydroelectric production. However, while tapping into the potential of tidal power seems simple, actual conditions present many challenges. Conventional hydroelectric technology (Figure 2) is not well suited to installation in Delta Works sluices. “The most common design for water turbines is the Kaplan-type rotor,” explains Van Halewijn. “It looks like the propeller used to power a ship. It turns very fast and if you place it in a confined space, like the sluices in our dams, it could damage fish and other sea life,” he says. To address these issues, Water2Energy has instead developed a vertical-axis water turbine (VAWT) that incorporates a Darrieus-type rotor (Figure 3).
Named after Georges Jean Marie Darrieus, who patented the Darrieus rotor for wind turbine use in 1926, this design offers potential benefits for water applications as well. From Water2Energy’s perspective, the most significant advantage of a Darrieus rotor is that its open structure and motion would be far less dangerous to fish than that of a Kaplan-type rotor. Would it be able to meet the ambitious power production goals of the ENCORE project? To balance the need to maximize electrical output while minimizing ecological harm, a number of challenges inherent to the Darrieus design had to be addressed.
Go with the Flow: Turbine Blades Self-Optimize Their Angle of Attack
For Water2Energy’s tidal power turbine, the most significant design decisions involved optimization of the rotor’s vertical blades (Figure 4). By testing and refining both the design of the blades and a mechanism to adjust their angle, Van Halewijn tackled two technical challenges. First, a Darrieus rotor is not always self-starting, even in an environment where water flows continuously. The second challenge? A spinning turbine installed in a contained passage, such as a sluice through a dam, is subject to more turbulence than one that spins freely in open air or water.
Both of these challenges can be met by continuously adjusting the turbine blades’ angle of attack. With the correct orientation toward water flow, a Darrieus rotor’s blade will start moving even at very low water speeds. The problem is that the optimal angle for starting the blade will be inefficient once the turbine is already moving. Similarly, a blade’s angle can be optimized to move smoothly past the wall of its enclosure, but that angle will be inefficient when the blade is at other points of its rotation. Van Halewijn used the COMSOL Multiphysics® software to model the effects of different blade positions on performance.
“Up to now, there has been no optimal design solution for this application. Water2Energy had some ideas for how to do it, and together with our simulation, we came to an even better solution,” says Van Halewijn. “We were able to test several ideas in the software to demonstrate an optimal approach."
To do so, Van Halewijn modeled just one blade of the turbine in order to find the optimal angle of attack. To model the turbulent flow around the turbine blade, he tried out different computational methods for fluid flow in the COMSOL® software. The standard k-ε model was not well-suited for the problem and did not lead to an optimal power output. The so-called SST model combines the k-ε model in the free stream and the k-ω model close to the walls, which led to good results, but made the model take too long to converge. Therefore, the k-ω model suited both the needs of the project balanced with the computational resources.
After modeling the turbulent flow around the turbine blade, Van Halewijn was able to find an optimal blade profile for the project. “I always explain to customers that simulation software is a decision tool for research and development. I am not selling mathematics. With simulation, I am able to move a project in the right direction, without as much trial and error. Really, what I am providing is better decisions based on the sound principles of physics," says Van Halewijn.
“Once we had modeled a profile for the blade, we could run simulations of its motion past the walls of the channel (Figures 5, 6). This meant we had to adjust the mesh of the blade’s surface to account for every step of its 360 degrees of rotation,” Van Halewijn explains. “I was able to add a special note in the software to maximize energy generation in the design phase. And of course, we had to simulate the passage of fish through the turbine, to convince people that sea life would not be harmed, not even by our prototype testing.”
Efficient and Fish Friendly: Live Tests Confirm New Design’s Potential
Water2Energy performed live testing of its adjusting-blade VAWT mechanism inside a Delta Works sluice channel (Figure 7). The tests demonstrated that, in terms of power output, the redesigned turbine outperformed an existing fixed-blade design by more than 40%.
Just as importantly, the Darrieus rotor turbine proved that it could turn tidal currents into electricity while protecting sea life. In turbines using Kaplan rotors, up to 20% of the fish flowing past it are typically killed by the fast-spinning blades. The Water2Energy turbine, as optimized by Van Halewijn’s modeling efforts, reduced the mortality rate to less than 1%. Cameras installed in the sluice channel vividly show the vertical adjusting blades working as intended, as trout swim safely past.
Having established the efficacy of their design, Water2Energy is now working to commercialize its potential. A consortium called Climate Power Plant Zeeland proposes to build a tidal power plant inside Zeeland’s Grevelingendam. One of the solutions proposed could use up to 6 Water2Energy turbines, totaling 1.6 MW of output, to generate electricity for an estimated 1000 households.
A Poetic (But Pragmatic) Engagement with the Rising Sea
Although he is happy to share the particulars of his tidal power turbine project, Van Halewijn also reminds us to take a broader view: “This story is not just about simulation,” he says. “You have to put it in the context of the problems we face nowadays.”
From this perspective, we can see the larger significance of this work being done by small companies in a small country. The wellbeing of the world may now depend on our ability to negotiate with natural forces, whether those forces are as vast as a North Sea storm, or as small — but significant, and even inspiring — as a trout swimming safely out with the tide. Says Van Halewijn, “We are looking for a win–win situation.”
Acknowledgments
The review of this article by Peter Scheijgrond from BLUESPRING is gratefully acknowledged.
About the ENCORE Project
The aim of the ENCORE project is to advance four offshore renewable energy technologies — a wave energy convertor, a tidal and river current turbine, and offshore floating solar — in a structured and collaborative process, and to develop open-source tools and services to facilitate the accelerated uptake of offshore energy solutions for islands, harbors, estuaries, and offshore structures with a focus on the Interreg 2 Seas region and export opportunities.
The ENCORE project receives funding from the Interreg 2 Seas program 2014–2020, cofunded by the European Regional Development Fund under subsidy contract No 2S08-004. Also, the provinces of South and North Holland and Zeeland are offering financial support.
Lead partner and coordinator BLUESPRING brings together project partners from 4 European countries: Water2Energy (NL), EEL Energy (FR), Oceans of Energy (NL), Teamwork Technology (NL), Dutch Marine Energy Centre (NL), the European Marine Energy Centre (UK), Artelia (FR), Bureau Veritas (FR), Ghent University (BE), Inyanga (UK), and Deftiq (NL).
Editor's note: This article was updated on February 7, 2023, to reflect organization name changes.
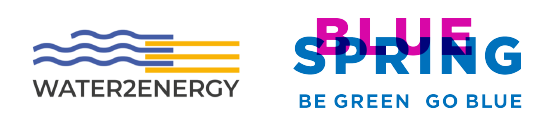
Download
- Physixfactor_2 Slides.pptx - 6.08MB
- Physixfactor_1 Slide.pptx - 5.95MB