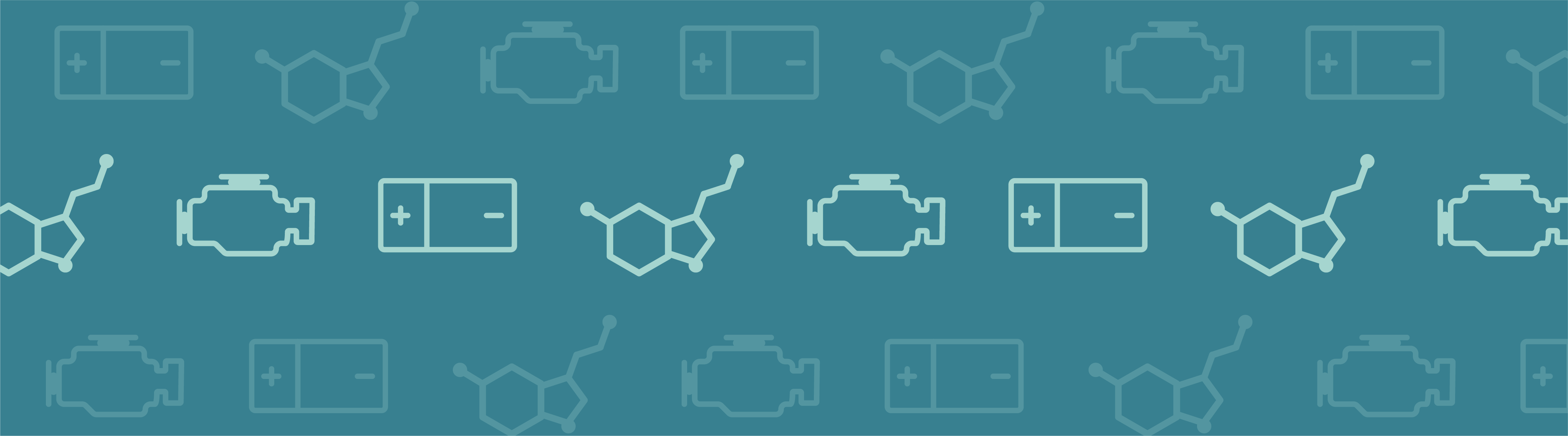
In an operating nuclear reactor, complex and highly coupled physical phenomena occur. Analyzing such phenomena within these devices by conducting physical experiments is often difficult and sometimes impossible. Simulation offers a simplified approach to studying and optimizing nuclear reactor designs, saving time, money, and other resources.
The Dynamics of a Nuclear Reactor
Let’s begin by looking at the mechanisms behind energy production in nuclear reactors. In these devices, energy is produced through a process known as fission. When the nucleus of a heavy atom — such as U-235, an isotope of uranium — absorbs a neutron, it splits into two lighter elements, or fission products. This process releases a large amount of kinetic energy, in addition to gamma radiation and free neutrons, which helps facilitate more fission events. When the different types of energy are converted to thermal energy, temperatures inside the reactor can reach around 1600 K.
Managing this heat requires the use of a coolant. The coolant, either water at high pressure or liquid metal, is circulated through the reactor core to remove excessive heat, as illustrated in the figure below. The branch of nuclear thermal hydraulics works with the flow of coolants and associated phenomena, which include the following:
- Heat transfer: Conduction, convection, and radiation
- Mass transfer: Phase change
- Fluid flow: Multiphase and turbulent flow
Fluid-structure interaction (FSI) is another important consideration within the reactor’s operating cycle. As the coolant flows through the reactor’s fuel plates, it causes the deflection of these components. Additionally, the large amount of heat that is generated can induce thermal stresses in the structural components of the reactor.
Schematic of a typical nuclear operating cycle. Image by Robert Steffens, via Wikimedia Commons.
Once the nuclear cycle is finished, the spent fuel is stored in containers and kept isolated in geological repositories. Using steel drums for waste storage brings the element of corrosion into the picture. Designing such repositories can be quite challenging, as the structures are excavated deep (300 m to 1000 m) into a stable geological environment. It is also important to ensure that the stored fission products, which have a half-life of hundreds of years, do not diffuse through the container and enter the groundwater supply.
Simplifying the Analysis of Nuclear Reactor Designs with Simulation
For nuclear reactors to operate safely and efficiently, it is critical that the phenomena described above are well understood. While some elements can be studied experimentally, performing tests under the harsh conditions within the reactor, such as high temperature and pressure levels and radioactive decay, can be rather difficult. And in cases like the analysis of radionuclide transport, which happens over a period of hundreds of years, experimental studies on actual time scales are nearly impossible to conduct.
Where experimental tests pose challenges, simulation can offer solutions — all while saving time and money. Given the coupled nature of nuclear reactors, COMSOL Multiphysics simulation software serves as an ideal platform for modeling the phenomena within these devices. Nuclear organizations across the world have used the software’s built-in features to analyze and design induction furnaces, electromagnetic pumps for liquid metal coolants, and RF cavities for accelerators, as well as for the nondestructive testing of reactor components.
Take Oak Ridge National Laboratory (ORNL), for instance. ORNL used COMSOL Multiphysics to improve their design of a major High Flux Isotope Reactor (HFIR). Using a simulation-based approach, the team was able to study the performance of the reactor’s components and the physics behind its operation, including thermal stresses, nonisothermal turbulent flow, and FSI. Researchers at ORNL also used simulation to support their efforts for safely converting HFIR to LEU fuel, a process that helped identify potential issues like buckling and hot spots within the design.
Simulation analyses enabled ORNL to study deflection in fuel plates, one of the primary components within a nuclear reactor’s core.
What about the challenge we mentioned earlier in regards to studying radionuclide transport experimentally? A team of researchers at the Swiss Nuclear Safety Inspectorate (ENSI) have modeled this phenomenon using COMSOL Multiphysics, too. The results from their study have been benchmarked against traditional safety codes for modeling radionuclide transport through clay barriers in a nuclear repository.
Advancing the Nuclear Industry
Simulation provides solutions to the challenges associated with studying nuclear reactors experimentally. Such an approach offers a more detailed and accurate look at the phenomena occurring within these devices, generating a deeper understanding of how to optimize their configuration. This insight paves the way toward developing safer and more efficient nuclear reactors, all while reducing the costs and resources associated with building prototypes to test each new design.
To learn more about the use of COMSOL Multiphysics in nuclear-based applications, check out the following resources:
- Researching a New Fuel for the HFIR: Advancements at ORNL Require Multiphysics Simulation to Support Safety and Reliability
- Battling Corrosion in Nuclear Waste Storage Facilities
- Carbon Steel Pipeline Wall Thickness Measurement Using Pulsed Eddy Current Technique
- Numerical Simulations of Radionuclide Transport through Clay and Confining Units in a Geological Repository using COMSOL
Comments (1)
ibrahim mohamed
July 21, 2019Thanks ..for sharing very helpful article